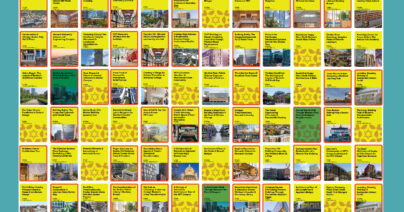
30 Acentech projects will be toured during AIA25 Conference!
Acentech worked on an astounding 30 projects that are being toured at the AIA25 Conference on Architecture in Boston next month! We had a great time…
“In Space, No One Can Hear You Scream.” That was the tagline for Ridley Scott’s 1979 sci-fi/horror masterpiece Alien, and this is certainly true for the fictional crewmembers in the proximity of a moon known as LV-426 situated 39 light-years from earth. It is also true for NASA astronauts Robert Behnken and Douglas Hurley, who recently joined the Expedition 63 crew 250 miles above Earth’s surface aboard the International Space Station (ISS). You see, sounds travel as molecules bump into each other. Think of a sound wave like an organized chain of bumper cars colliding into one another, traveling through the earth’s atmosphere, sending information from my mouth to your ears. When we remove all the bumper cars (or molecules in the earth’s atmosphere), there is no medium to transfer these collisions, and this means sound waves cannot propagate. Acoustic waves require a medium to travel through, and in the vacuum of outer space, there are simply not enough molecules packed densely enough to propagate sound waves as we know them. But are there or have there ever been parts of space where acoustic waves can propagate? Yes, and the reason why may surprise you.
To find acoustic waves in outer space, we must ask ourselves two questions: When? And Where? In part 1 of our journey, we will explore the when of sound in space.
How did the universe begin? Our best bet is it started as an initial singularity. As far as we know, all the mass and space-time of the universe likely existed within a single, infinitesimally small point. You may have heard what happened next. The universe started off with a bang – a Big Bang – generating immense heat and bursting into existence an intimate fluid of photons (light), electrons, and other fundamental particles. All this energy and matter needed somewhere to go, and the universe began to expand from its singular origin. Once the universe expanded enough, temperatures began to stabilize, baryogenesis created a liking of matter over antimatter, and elementary particles (quarks and leptons) began to form composite particles such as protons and neutrons. Early universe nuclei formed from protons and neutrons only a few minutes after the Big Bang.
Things were still too hot to complete the simplest neutral atoms; electrons could not bind to atomic nuclei and the hydrogen and helium gasses present were fully ionized. Rogue electrons whizzed through the primordial soup, bumping into photons and scattering light to create a bright, opaque fog that permeated the entire universe. Eventually as the universe expanded and cooled down more, around 400,000 years after the Big Bang, electrons became able to bind with nuclei and the first real atoms formed in a period known as the recombination epoch. Without the immense heat and scattering of light caused by unbound electrons, the light dispersed, and the fog of the universe quickly cleared. We could now see our newly vacuous cosmic surroundings.
Diverging from sound for a moment, to understand what is to come, you must know two things about light. First, light can propagate on its own. It does not need a medium to propagate through as sound does. Light does not need the bumper cars we discussed earlier. Second, light travels at a fixed, finite speed. This fixed speed happens to be the fastest speed anything can travel in the universe. It, therefore, takes a fixed amount of time for light to travel a fixed distance. A light-year is actually a measure of distance; it is exactly how far a photon will travel in a year. When a photon travels to you from a location one light-year away, what you observe will be that location one year in the past. So, when you look out over large distances in space, you are actually looking through time.
Remember how we said the universe was once full of a hot fog of light and ionized gas? Well, if we look far enough into deep space, we can actually look back in time long enough to see when the hot primordial fog faded, the recombination epoch began, and our surroundings became visible. As we look out into the deepest chasms of space in all directions, we see this fog wall appear. The light that was once bouncing about in a sea of rogue electrons and nuclei was now able to travel freely through space as atoms formed. Photons decoupled from their scattering prison and raced from this pre-atomic fog wall to our eyes (or telescopes) here on earth. Many physicists believed that because the universe is uniform on large scales, the light reaching the earth from this era would arrive with equal intensity from all directions.
When we take a closer look into the past at this fog wall, we do not see the homogeneity in the pre-atomic universe as many had predicted. We see patchiness. How do we know all this? We have a map: The Cosmic Microwave Background.
Disregarding the fact that NASA’s Wilkinson Microwave Anisotropy Probe (WMAP) heat map took 9 years to make and isn’t even our most accurate survey of the CMB, it is a useful visual indicating how hot any given place was when the electron-induced photon fog wall disappeared and atoms materialized. It is essentially a snapshot of the last state the universal fog was in before it lifted.
The non-uniform nature of this map is caused by differences in density, temperature, and pressure at different locations on the map. One of the proposed mechanisms for generating this imbalance is acoustic waves. Why acoustic waves? Well, we know acoustic waves create differences in density, temperature, and pressure here on earth. They create zones of compression where air molecules are densely packed and zones of rarefaction where air molecules are more spread out. The more particles you have in a given space, the denser the space becomes, and the hotter it reads in the CMB snapshot.
“But I thought acoustic waves couldn’t propagate in space. There is no atmosphere, as you already explained!” You are correct that, currently, sound waves audible to our ears are not present in outer space; Ridley Scott was correct in his trailer for Alien. However, this was not always the case. Due to the expanding of space over time caused by the Big Bang, the universe was around 1,000 times smaller than it currently is around the time the fog wall lifted. This early universe’s primordial soup had all the energy and matter we know exists today in a much smaller space; a space compact enough to form an atmosphere (of sorts), and dense enough to allow for acoustic waves to propagate. This universal “atmosphere” was about a billion times denser than outer space’s average density today. Much like how the earth’s atmosphere introduces the bumper cars for us to hear things like speaker arrays, human speech, and symphonies, this primordial “atmosphere” was dense enough that it allowed sound waves to propagate.
We visualize acoustic waves in performing arts centers in much the same way that the CMB snapshot visualizes acoustic waves in the early universe. Both are color-coded maps theorized to be the result (in part) of acoustic waves. One measures sound pressure levels in decibels and the other temperature in microKelvin. One is room-sized, one is cosmically large.
For starters, the Big Bang itself was silent. Physicists believe everything started out relatively uniform, and there were no fluctuations in pressure, temperature, or anything. Because sound waves rely on the relative motion between particles, there was no sound originally. More on this to come in part 2 (See On the Origins of Sound). What we can say is sound was generated as the universe inflated from a singularity, and by the time recombination occurred, there were acoustic waves.
So, what if we want to jump 400,000 years after the Big Bang and analyze the Cosmic Microwave Background like it is a sound map? We know there could have been acoustic waves present, and we want to learn more about these waves. With some laborious calculations, we can determine the pressure needed to cause each patch of raised or lowered temperature present in the CMB and compare that to the ambient temperature and pressure of the primordial universe as a whole. We want to study small pressure fluctuations in the same way we do when we study sound waves here on Earth.
To determine very roughly how loud these temperature patches are in decibels, we need to develop a reference pressure. We will use the same reference pressure scale commonly used to measure sounds in air. This is on the order of 0.2 billionths of the typical atmospheric pressure and would read 0 dB when measured. Comparing a root mean square (RMS) pressure to a reference pressure is the technique microphones use to determine sound pressure levels in concert halls, on construction sites, and in football stadiums.
So what is the RMS pressure? Assuming temperature and pressure are proportional, we can compare the tiny pressure fluctuations that display themselves at patches in the CMB to the overall ambient pressure of the entire early universe “atmosphere.” The root mean square (rms) pressure variation of the CMB is around 10 millionths of the typical CMB ambient pressure. Since we have a rough idea of the RMS pressure and reference pressure of the CMB, we can actually calculate a decibel level for the early universe, essentially telling us how loud the early universe was! The early universe likely experienced sound levels upwards of 120 dB. This is about as loud as an electronic dance music concert or a jet taking off; though keep in mind rock concerts and jets use a much more earth-friendly reference pressure. The overall magnitudes of these cosmic acoustic waves are quite different from any acoustic waves produced on earth, but their size in comparison to the ambient pressure around them is similar.
Acousticians want to know about frequency content in addition to overall sound levels. If the early universe had sound waves like those on earth, what frequencies did the Big Bang produce? Here on earth, we can measure the frequency of sound waves by counting how much time passes between wave peaks. For example, a 60 Hz tone will produce pressure peaks roughly once every 0.017 seconds, or 60 peaks in one second. More complex tones arise from things like flutes, which produce a series of harmonics shown as the sharp peaks below.
Due to the immense cosmic size of the early universe, we cannot simply measure the time between wave peaks in the cosmic microwave background. The periods of primordial acoustic waves are somewhere between 20,000 to 200,000 years! Since the CMB was only discovered in 1964, we need to find a different way to measure wave frequency. We will explore this topic and more in Cosmic Acoustics: Part II.